The dielectric profile of water at solid surfaces and the origin of the Stern layer
Electrostatic interactions between charged objects in water, such as
lipid membranes, proteins and ions, are profoundly influenced by the
polarity of the water.
In a macroscopic approach, the effect of the water on electrostatic
interactions is quantified by means of the static dielectric tensor,
which is spatially constant and diagonal in bulk.
Close to an interface, however, the effect of the water is more
intricate.
Using atomistic molecular dynamics simulations, we calculate the
space-dependent dielectric response function of water at solid surfaces.
The proximity of a surface induces spatial ordering and orientation in the first few nanometres of water. Because of the very polar nature of water molecules, this water structure directly affects the electrostatic environment, making the dielectric tensor inherently space-dependent.
The dielectric function of water in thin interfacial layers strongly affects macromolecular interactions because of the abundance of interfacial water at small length scales. Therefore, the dielectric function is of great interest, both in the field of colloid and biological physics, and in the view of nano-technological applications. Not only can our results help understanding fundamental biological interactions, they can also provide a guide for the design of industrial applications like high power density batteries based on the double layer capacitance. As the modified dielectric properties at interfaces are traditionally contained in the so-called Stern layer, this work provides a firm theoretical footing for the Stern layer concept.
The proximity of a surface induces spatial ordering and orientation in the first few nanometres of water. Because of the very polar nature of water molecules, this water structure directly affects the electrostatic environment, making the dielectric tensor inherently space-dependent.
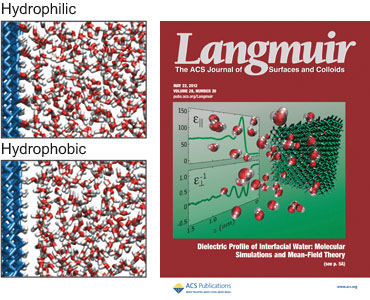
Fig. 1.
Images of the molecular structure of hydrophilic and hydrophobic diamond/water interfaces in molecular dynamics simulations.
Cover image source: American Chemical Society.
At planar surfaces, the dielectric tensor has two unique components, one
parallel and one perpendicular to the interface.
We calculate both components at hydrophilic and hydrophobic diamond
surfaces (Fig. 1) using atomistic molecular dynamics simulations [1,2].
Whereas the parallel component roughly follows the water density (Fig.
2a-b) — as expected for non-interacting polar molecules — the
perpendicular component has a radically different structure (Fig. 2c-d).
The curve of the inverse perpendicular component goes through zero
several times, which
means that the dielectric response exhibits singularities and
overscreening.
Interestingly, we find that the quadrupole and higher order electric
multipoles contribute significantly to the dielectric response.
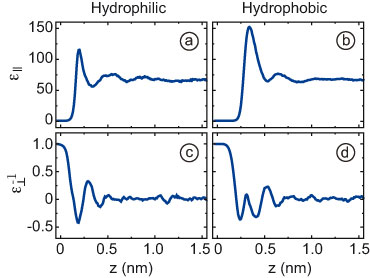
Fig. 2.
The parallel (a,b) and inverse perpendicular (c,d) dielectric response
functions of water next to a hydrophilic (a,c) and a hydrophobic (b,d)
diamond surface, calculated from atomistic molecular dynamics
simulations.
To study the effect of the dielectric environment on macroscopic length
scales, we incorporate the perpendicular component of the dielectric
tensor in mean-field continuum theory.
In particular, this strategy allows us to calculate the double layer
capacitance, which is formed by a charged surface and its counterion
cloud.
The calculated capacitance as a function of bulk salt concentration
agrees exceptionally well with experimental results [1].
We also study the effect on surface forces [2], ion distributions [3] and ion solvation energies [4].
The dielectric function of water in thin interfacial layers strongly affects macromolecular interactions because of the abundance of interfacial water at small length scales. Therefore, the dielectric function is of great interest, both in the field of colloid and biological physics, and in the view of nano-technological applications. Not only can our results help understanding fundamental biological interactions, they can also provide a guide for the design of industrial applications like high power density batteries based on the double layer capacitance. As the modified dielectric properties at interfaces are traditionally contained in the so-called Stern layer, this work provides a firm theoretical footing for the Stern layer concept.
[1] | D. J. Bonthuis, S. Gekle and R. R. Netz; Dielectric profile of interfacial water and its effect on double-layer capacitance; Phys. Rev. Lett. 107, 166102 (2011). |
[2] | D. J. Bonthuis, S. Gekle and R. R. Netz; Profile of the static permittivity tensor of water at interfaces: Consequences for capacitance, hydration interaction and ion adsorption; Langmuir 28, 7679 (2012). |
[3] | D. J. Bonthuis and R. R. Netz; Beyond the continuum: How molecular solvent structure affects electrostatics and hydrodynamics at solid-electrolyte interfaces; J. Chem. Phys. B 117, 11397 (2013). |
[4] | D. J. Bonthuis and R. R. Netz; Dielectric profiles and ion-specific effects at aqueous interfaces; In "Electrostatics of Soft and Disordered Matter", pages 129-142, Pan Stanford Publishing (2014). |